Research overview
For a long time, it was unclear how animal lifespan and aging are determined, but recent studies using model organisms have shown that lifespan and aging are clearly regulated processes. So far, several molecular mechanisms, so-called lifespan extension pathways such as reduced insulin/IGF-1 signaling, dietary restriction, germline removal and reduced mitochondrial respiration have been identified. Interestingly, an intracellular bulk degradation system, autophagy-lysosomal pathway is commonly activated in all these longevity pathways and its function is critical for lifespan extension (Fig. 1). On the other hand, it has been shown that the activity of the autophagy-lysosomal pathway declines with age, and this is one of main causes for aging and age-related diseases. These findings suggest that this autophagy-lysosomal pathway is a convergent key process to control animal lifespan and ageing. However, many fundamental questions remain unanswered such as its regulatory mechanisms and actual roles in the regulation of lifespan and aging. By utilizing several models such as mammalian cultured cells, yeast (S. cerevisiae), worm (C. elegans) and mice, we aim to uncover the mystery of aging through understanding the molecular and cellular mechanism of autophagy-lysosomal pathway.
On going projects:
- Mechanisms of lifespan extension and suppression of aging by the autophagy-lysosomal pathway
- Mechanisms of age-related dysfunction of the autophagy-lysosomal pathway
- Molecular mechanisms of lysosomal homeostasis and its age-related changes
- Molecular mechanisms responsible for the trade-off between reproduction and lifespan
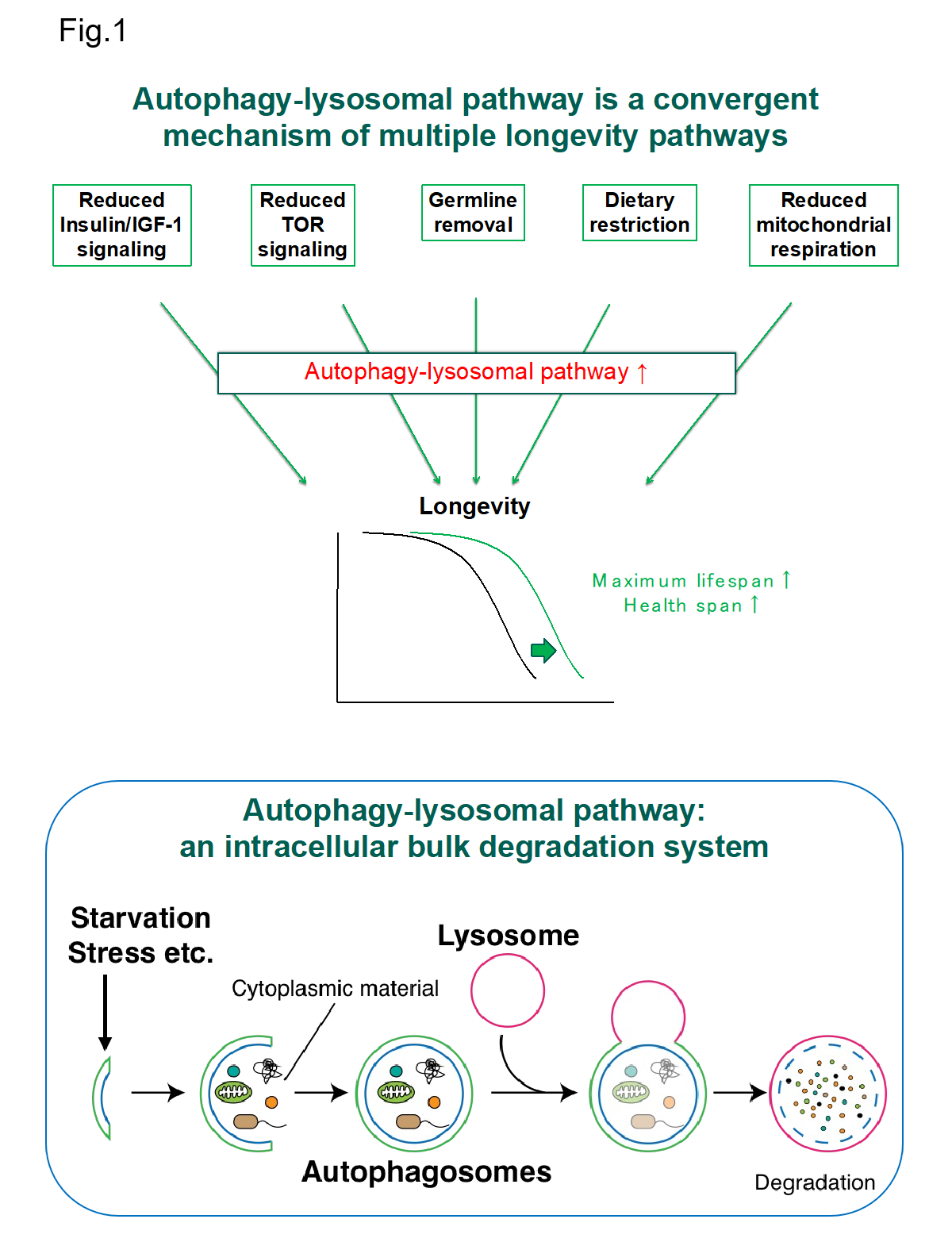
Recent key publications
- Cui, M., Yamano, K., Yamamoto, K., Yamamoto-Imoto, H., Minami, S., Yamamoto, T., Matsui, S., Kaminishi, T., Shima, T., Ogura, M., Tsuchiya, M., Nishino, K., Layden, B., Kato, H., Ogawa, H., Oki, S., Okada, Y., Isaka, Y., Kosako, H., Matsuda, N., *Yoshimori, T., *Nakamura, S. HKDC1, a target of TFEB, is essential to maintain both mitochondrial and lysosomal homeostasis, preventing cellular senescence. PNAS 121(2), (2024)
- Ogura, M., Kaminishi, T., Shima, T., Torigata, M., Bekku, N., Tabata, K., Minami, S., Nishino, K., Nezu, A., Hamasaki, M., Kosako, H., *Yoshimori, T., *Nakamura, S. Microautophagy regulated by STK38 and GABARAPs is essential to repair lysosomes and prevent aging. EMBO Rep., e57300, (2023).
- Shioda, T., Ikenaka, K., Fujita, N., Kanki, T., Oka, T., Mochizuki, H., Antebi, A., *Yoshimori, T., *Nakamura, S. Neuronal MML-1/MXL-2 regulates systemic aging via glutamate transporter and cell non-autonomous autophagic and peroxidase activity. PNAS, 120, e2221553120, (2023).
- Oe, Y., Kakuda, K., Yoshimura, S., Hara, N., Hasegawa, J., Terawaki, S., Kimura, Y., Ikenaka, K., Suetsugu, S., Mochizuki, H., *Yoshimori, T., *Nakamura, S. PACSIN1 is indispensable for amphisome-lysosome fusion during basal autophagy and subsets of selective autophagy. PLoS Genet., 18(6), e1010264, (2022)
- Yamamoto-Imoto, H., Minami, S., Shioda, T., Yamashita, Y., Sakai, S., Maeda, S., Yamamoto, T., Oki, S., Takashima, M., Yamamuro, T., Yanagawa, K., Edahiro, R., Iwatani, M., So, M., Tokumura, A., Abe, T., Imamura, R., Nonomura, N., Okada, Y., Ayer, E D., Ogawa, H., Hara, E., Takabatake, Y., Isaka, Y., *Nakamura, S., *Yoshimori, T. Age-associated decline of MondoA drives cellular senescence through impaired autophagy and mitochondrial homeostasis. Cell Rep., 38(9): 110444, (2022)
- Yamamuro, T., *Nakamura, S., Yamano, Y., Endo, T., Yanagawa, K., Tokumura, A., Matsumura, T., Kobayashi, K., Mori, H., Enokidani, Y., Yoshida, G., Imoto, H., Kawabata, T., Hamasaki, M., Kuma, A., Kuribayashi, S., Takezawa, K., Okada, Y., Ozawa, M., Fukuhara, S., Shinohara, T., Ikawa, M., *Yoshimori, T. Rubicon prevents autophagic degradation of GATA4 to promote Sertoli cell function. PLoS Genet., 17(8), e1009688, (2021)
- Fujita, T., Kubo, S., Shioda, T., Tokumura, A., Minami, S., Tsuchiya, M., Isaka, Y., Ogawa, H., Hamasaki, M., Yu, L., *Yoshimori, T., *Nakamura, S. THOC4 regulates energy homeostasis by stabilizing TFEB mRNA during prolonged starvation. J. Cell Sci.,134(6): jcs248203, (2021)
- *Nakamura, S., Shigeyama, S., Minami, S., Shima, T., Akayama, S., Matsuda, T., Esposito, A., Napolitano, G., Kuma, A., Namba-Hamano, T., Nakamura, J., Yamamoto, K., Sasai, M., Tokumura, A., Miyamoto, M., Oe, Y., Fujita, T., Terawaki, S., Takahashi, A., Hamasaki, M., Yamamoto, M., Okada, Y., Komatsu, M., Nagai, T., Takabatake, Y., Xu, H., Isaka, Y., Ballabio, A., *Yoshimori, T. LC3 lipidation is essential for TFEB activation during the lysosomal damage response to kidney injury. Nat. Cell Biol., 22(10): 1252-1263, (2020)
- Nakamura, S., Oba, M, Suzuki M, Takahashi T, Yamamuro T, Fujiwara M, Ikenaka K, Minami M, Tabata N, Yamamoto, K., Kubo, S., Tokumura A., Akamatsu, K., Miyazaki, Y., Kawabata, T., Hamasaki, M., Fukui, K., Sango, K., Watanabe, Y., Takabatake, Y., Kitajima, S T., Okada, Y., Mochizuki, H., Isaka, Y., Antebi, A., *Yoshimori, T. Suppression of autophagic activity by Rubicon is a signature of aging. Nat Commun.,10(1): 847, (2019)
- Nakamura, S., Karalay, O., Jaeger, P, Horikawa, M., Nakamura, K., Latza, C., Klein, C., Templer, S., Dieterich, C., *Antebi, A. Mondo complexes regulate TFEB via TOR inhibition to promote longevity in response to gonadal signals. Nat Commun.,7: 10944, (2016)